Simulation of a Novel Induced-Charge Electrokinetic Actuation Mechanism for a Diaphragm Micropump
From microelectronics cooling to biological analysis and wearable therapeutic infusion systems, micropumps are steadily becoming a valuable and ubiquitous tool for fluid transport and thermal management. Hence, the development of novel actuation mechanisms that allow for precise manipulation of small fluid volumes is of high interest and great utility. To date, commercialization efforts have largely been centered around piezoelectric, mechanical, and electrochemical micropumping methods. While these techniques have proven effective at moving relatively “large” volumes of fluid (μL - mL), fabrication constraints and a number of other hurdles have limited the development of technology for more precise manipulation of very small (nL - μL) amounts of fluid; such processes are in increasingly high demand within the emerging field of miniaturized and integrated bioanalytical systems (e.g. wearable biosensors), for example. To address this need, we introduce a novel electrokinetic actuation technique for a reciprocating positive-displacement micropump. Our proposed mechanism leverages internally-generated pressure gradients arising from induced-charge electroosmotic flow (ICEOF) at a micro/nanochannel-confined bipolar electrode (BPE) to controllably deform a flexible diaphragm and displace fluid in an adjacent channel. No electrochemical reactions at the BPE are necessary, though increased operating pressures from hybrid hydrodynamic-electrochemical actuation can potentially improve the pumping throughput. Moreover, a highly desirable feature of employing BPEs is that they provide “wireless” actuation - as opposed to conventional systems which require internal circuitry to address each element, the electrolyte solution serves as the conductive path in a BPE system. Thus, any number of isolated BPE elements operating in parallel can be “activated” for pumping using a single applied voltage difference between two driving electrodes connected to a fluidic channel array.
We demonstrate the operating principle by numerically modeling the highly coupled Electrostatics (AC/DC Module), Chemical Species Transport (Microfluidics Module), Hydrodynamics (Microfluidics Module), and Solid Mechanics (Structural Mechanics Module) equations in 2D using COMSOL Multiphysics® simulation software. By resolving the diffuse charge layer and accounting for capacitive effects of the compact layer, our model is capable of capturing the impact of surface polarization and ionic charge screening dynamics on the nonlinear electrokinetics and resulting ICEOF. Moreover, our model allows for Faradaic current injection and charge accumulation by redox reactions occurring at the BPE poles. The highly coupled nature of the numerically stiff electrokinetic transport equations and the deforming geometry from the fluid-structure interactions leads to computation times of up to three days for transient actuation studies; to improve time and computational efficiency, we have therefore developed an additional simplified model which we employ for parametric studies into salient design considerations such as system lengths, heights, and diaphragm construction. Using these two models, we show that the integration of polymer flaps to act as passive valves enables continuous, unidirectional pumping for a wide range of flow rates (pL/s – μL/s) by simply applying a sinusoidal potential difference across the actuation channel (see figure). This BPE-induced reciprocating micropumping mechanism provides a promising approach for the low-power, precise manipulation of small fluid volumes that can be spatially and electrochemically isolated from contamination.
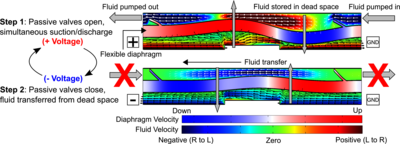
Download
- eden_poster.pdf - 3.5MB