Na-MCl2 Cell Multiphysics Modeling: Status and Challenges
Introduction: After more than 20 years of experience in EV applications, the sodium nickel chloride technology is fully mature for large scale energy storage. Early on, mathematical modeling of Na-MCl2 cell (M standing for Fe or Ni) has attracted attention to help identifying predominant mechanisms at work during operation. However, these models were rarely validated with data coming from commercial cells. The first aim of this talk is to present a state of the art of multiphysics models that have been published so far for this technology, including our own contribution regarding the cell manufactured by FIAMM. We will also discuss the implementation of new modeling hypotheses that have emerged from recent in situ experimental analyses [1-2].
Use of COMSOL Multiphysics®: All the mathematical battery models detailed in this work [3-6], including ours, have been derived from the seminal paper published by Sudoh and Newman in 1990 [7]. COMSOL Multiphysics® software has been used to extend this 1D model (of an iron based cell) to a comprehensive 2D model, relying on the exact geometry of the commercial cell, and its material content (Ni/Fe mixed-cell). In the model, materials are defined by some key temperature dependent properties (conductivity, density...).
Electrochemical processes and volume fraction changes are described through various PDE and ODE, and model variables (porosity, local transfer current...). Subsequently, thermal coupling has been implemented via the Heat Transfer in Solids physics interface. Besides, the ability of COMSOL to parameterize the geometry has been of great interest to predict the impact of design on the overall cell performance.
Results: Testing the previous models in COMSOL helped us determining the relevant mechanisms to implement in our model. A sensitivity analysis was then performed to focus our work on parameters that have the most significant impact on the simulation results. Finally, the model has been successfully validated. Figure 1 displays a comparison between the simulated (black) and experimental (blue) voltages of the cell during a discharge.
This is the first time one tries to simulate a discharge featuring both contributions of Ni (first plateau beginning at 2.5 V) and Fe (second plateau beginning at 2.28 V) active materials. It is also worth mentioning that this simulation has been obtained with heat transfer coupling, and in accordance with analytical results published very recently [1-2]. However, implementation of the charge phases proved to be more challenging than a simple current inversion. Hypotheses regarding relaxation mechanisms during post discharge/charge phases are also discussed.
Conclusion: We demonstrate that our multiphysics model is suitable to simulate the electrochemical and thermal behaviors of a Na-MCl2 commercial cell, at various rates and from different initial temperatures. This model gives us a better understanding of the processes involved during the cell operation. It is sufficiently robust and versatile to improve the cell design and the active materials balance.
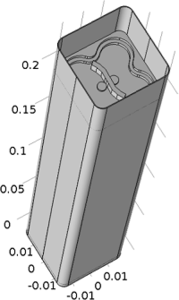
Download
- christin_presentation.pdf - 2.65MB
- christin_abstract.pdf - 0.09MB